Eutrophication

Part of a series on |
Plankton |
---|
![]() |
Eutrophication is a general term describing a process in which nutrients accumulate in a body of water, resulting in an increased growth of microorganisms that may deplete the oxygen of water.[1][2] Eutrophication may occur naturally or as a result of human actions. Manmade, or cultural, eutrophication occurs when sewage, industrial wastewater, fertilizer runoff, and other nutrient sources are released into the environment.[3] Such nutrient pollution usually causes algal blooms and bacterial growth, resulting in the depletion of dissolved oxygen in water and causing substantial environmental degradation.[4]
Approaches for prevention and reversal of eutrophication include minimizing point source pollution from sewage and agriculture as well as other nonpoint pollution sources.[1] Additionally, the introduction of bacteria and algae-inhibiting organisms such as shellfish and seaweed can also help reduce nitrogen pollution, which in turn controls the growth of cyanobacteria, the main source of harmful algae blooms.[5]
History and terminology
[edit]![]() | This section needs expansion. You can help by adding to it. (February 2024) |
The term "eutrophication" comes from the Greek eutrophos, meaning "well-nourished".[6] Water bodies with very low nutrient levels are termed oligotrophic and those with moderate nutrient levels are termed mesotrophic. Advanced eutrophication may also be referred to as dystrophic and hypertrophic conditions.[7] Thus, eutrophication has been defined as "degradation of water quality owing to enrichment by nutrients which results in excessive plant (principally algae) growth and decay."[8]
Eutrophication was recognized as a water pollution problem in European and North American lakes and reservoirs in the mid-20th century.[9] Breakthrough research carried out at the Experimental Lakes Area (ELA) in Ontario, Canada, in the 1970s provided the evidence that freshwater bodies are phosphorus-limited. ELA uses the whole ecosystem approach and long-term, whole-lake investigations of freshwater focusing on cultural eutrophication.[10]
Causes
[edit]
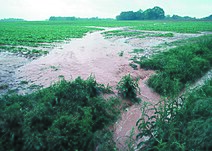
Eutrophication is caused by excessive concentrations of nutrients, most commonly phosphates and nitrates,[11] although this varies with location. Prior to their being phasing out in the 1970's, phosphate-containing detergents contributed to eutrophication. Since then, sewage and agriculture have emerged as the dominant phosphate sources.[12] The main sources of nitrogen pollution are from agricultural runoff containing fertilizers and animal wastes, from sewage, and from atmospheric deposition of nitrogen originating from combustion or animal waste.[13]
The limitation of productivity in any aquatic system varies with the rate of supply (from external sources) and removal (flushing out) of nutrients from the body of water.[14] This means that some nutrients are more prevalent in certain areas than others and different ecosystems and environments have different limiting factors. Phosphorus is the limiting factor for plant growth in most freshwater ecosystems,[15] and because phosphate adheres tightly to soil particles and sinks in areas such as wetlands and lakes,[16] due to its prevalence nowadays more and more phosphorus is accumulating inside freshwater bodies.[17][18] In marine ecosystems, nitrogen is the primary limiting nutrient; nitrous oxide (created by the combustion of fossil fuels) and its deposition in the water from the atmosphere has led to an increase in nitrogen levels,[19] and also the heightened levels of eutrophication in the ocean.[20]
Cultural eutrophication
[edit]Cultural or anthropogenic eutrophication is the process that causes eutrophication because of human activity.[21][22] The problem became more apparent following the introduction of chemical fertilizers in agriculture (green revolution of the mid-1900s).[23] Phosphorus and nitrogen are the two main nutrients that cause cultural eutrophication as they enrich the water, allowing for some aquatic plants, especially algae to grow rapidly and bloom in high densities. Algal blooms can shade out benthic plants thereby altering the overall plant community.[24] When algae die off, their degradation by bacteria removes oxygen, potentially, generating anoxic conditions. This anoxic environment kills off aerobic organisms (e.g. fish and invertebrates) in the water body. This also affects terrestrial animals, restricting their access to affected water (e.g. as drinking sources). Selection for algal and aquatic plant species that can thrive in nutrient-rich conditions can cause structural and functional disruption to entire aquatic ecosystems and their food webs, resulting in loss of habitat and species biodiversity.[25]
There are several sources of excessive nutrients from human activity including run-off from fertilized fields, lawns, and golf courses, untreated sewage and wastewater and internal combustion of fuels creating nitrogen pollution.[26] Cultural eutrophication can occur in fresh water and salt water bodies, shallow waters being the most susceptible. In shore lines and shallow lakes, sediments are frequently resuspended by wind and waves which can result in nutrient release from sediments into the overlying water, enhancing eutrophication.[27] The deterioration of water quality caused by cultural eutrophication can therefore negatively impact human uses including potable supply for consumption, industrial uses and recreation.[28]

Natural eutrophication
[edit]Eutrophication can be a natural process and occurs naturally through the gradual accumulation of sediment and nutrients. Naturally, eutrophication is usually caused by the natural accumulation of nutrients from dissolved phosphate minerals and dead plant matter in water.[29][30]
Natural eutrophication has been well-characterized in lakes. Paleolimnologists now recognise that climate change, geology, and other external influences are also critical in regulating the natural productivity of lakes. A few artificial lakes also demonstrate the reverse process (meiotrophication[31]), becoming less nutrient rich with time as nutrient poor inputs slowly elute the nutrient richer water mass of the lake.[32][33] This process may be seen in artificial lakes and reservoirs which tend to be highly eutrophic on first filling but may become more oligotrophic with time. The main difference between natural and anthropogenic eutrophication is that the natural process is very slow, occurring on geological time scales.[34]
Effects
[edit]
Ecological effects
[edit]Eutrophication can have the following ecological effects: increased biomass of phytoplankton, changes in macrophyte species composition and biomass, dissolved oxygen depletion, increased incidences of fish kills, loss of desirable fish species.[35]
Decreased biodiversity
[edit]When an ecosystem experiences an increase in nutrients, primary producers reap the benefits first. In aquatic ecosystems, species such as algae experience a population increase (called an algal bloom). Algal blooms limit the sunlight available to bottom-dwelling organisms and cause wide swings in the amount of dissolved oxygen in the water. Oxygen is required by all aerobically respiring plants and animals and it is replenished in daylight by photosynthesizing plants and algae. Under eutrophic conditions, dissolved oxygen greatly increases during the day, but is greatly reduced after dark by the respiring algae and by microorganisms that feed on the increasing mass of dead algae. When dissolved oxygen levels decline to hypoxic levels, fish and other marine animals suffocate. As a result, creatures such as fish, shrimp, and especially immobile bottom dwellers die off.[36] In extreme cases, anaerobic conditions ensue, promoting growth of bacteria. Zones where this occurs are known as dead zones.
New species invasion
[edit]Eutrophication may cause competitive release by making abundant a normally limiting nutrient. This process causes shifts in the species composition of ecosystems. For instance, an increase in nitrogen might allow new, competitive species to invade and out-compete original inhabitant species. This has been shown to occur in New England salt marshes.[37] In Europe and Asia, the common carp frequently lives in naturally eutrophic or hypereutrophic areas, and is adapted to living in such conditions. The eutrophication of areas outside its natural range partially explain the fish's success in colonizing these areas after being introduced.
Toxicity
[edit]Some harmful algal blooms resulting from eutrophication, are toxic to plants and animals.[21][38] Freshwater algal blooms can pose a threat to livestock. When the algae die or are eaten, neuro- and hepatotoxins are released which can kill animals and may pose a threat to humans.[39][40] An example of algal toxins working their way into humans is the case of shellfish poisoning.[41] Biotoxins created during algal blooms are taken up by shellfish (mussels, oysters), leading to these human foods acquiring the toxicity and poisoning humans. Examples include paralytic, neurotoxic, and diarrhoetic shellfish poisoning. Other marine animals can be vectors for such toxins, as in the case of ciguatera, where it is typically a predator fish that accumulates the toxin and then poisons humans.
Economic effects
[edit]Eutrophication and harmful algal blooms can have economic impacts due to increasing water treatment costs, commercial fishing and shellfish losses, recreational fishing losses (reductions in harvestable fish and shellfish), and reduced tourism income (decreases in perceived aesthetic value of the water body).[42] Water treatment costs can be increased due to decreases in water transparency (increased turbidity). There can also be issues with color and smell during drinking water treatment.
Health impacts
[edit]Human health effects of eutrophication derive from two main issues excess nitrate in drinking water and exposure to toxic algae.[43] Nitrates in drinking water can cause blue baby syndrome in infants and can react with chemicals used to treat water to create disinfection by-products in drinking water.[44] Getting direct contact with toxic algae through swimming or drinking can cause rashes, stomach or liver illness, and respiratory or neurological problems .[45]
Causes and effects for different types of water bodies
[edit]
Freshwater systems
[edit]One response to added amounts of nutrients in aquatic ecosystems is the rapid growth of microscopic algae, creating an algal bloom. In freshwater ecosystems, the formation of floating algal blooms are commonly nitrogen-fixing cyanobacteria (blue-green algae). This outcome is favored when soluble nitrogen becomes limiting and phosphorus inputs remain significant.[46] Nutrient pollution is a major cause of algal blooms and excess growth of other aquatic plants leading to overcrowding competition for sunlight, space, and oxygen. Increased competition for the added nutrients can cause potential disruption to entire ecosystems and food webs, as well as a loss of habitat, and biodiversity of species.[25]
When overproduced macrophytes and algae die in eutrophic water, their decompose further consumes dissolved oxygen. The depleted oxygen levels in turn may lead to fish kills and a range of other effects reducing biodiversity. Nutrients may become concentrated in an anoxic zone, often in deeper waters cut off by stratification of the water column and may only be made available again during autumn turn-over in temperate areas or in conditions of turbulent flow. The dead algae and organic load carried by the water inflows into a lake settle to the bottom and undergo anaerobic digestion releasing greenhouse gases such as methane and CO2. Some of the methane gas may be oxidised by anaerobic methane oxidation bacteria such as Methylococcus capsulatus, which in turn may provide a food source for zooplankton.[47] Thus a self-sustaining biological process can take place to generate primary food source for the phytoplankton and zooplankton depending on the availability of adequate dissolved oxygen in the water body.[48]
Enhanced growth of aquatic vegetation, phytoplankton and algal blooms disrupts normal functioning of the ecosystem, causing a variety of problems such as a lack of oxygen which is needed for fish and shellfish to survive. The growth of dense algae in surface waters can shade the deeper water and reduce the viability of benthic shelter plants with resultant impacts on the wider ecosystem.[24][49] Eutrophication also decreases the value of rivers, lakes and aesthetic enjoyment. Health problems can occur where eutrophic conditions interfere with drinking water treatment.[50]
Phosphorus is often regarded as the main culprit in cases of eutrophication in lakes subjected to "point source" pollution from sewage pipes. The concentration of algae and the trophic state of lakes correspond well to phosphorus levels in water. Studies conducted in the Experimental Lakes Area in Ontario have shown a relationship between the addition of phosphorus and the rate of eutrophication. Later stages of eutrophication lead to blooms of nitrogen-fixing cyanobacteria limited solely by the phosphorus concentration.[51] Phosphorus-base eutrophication in fresh water lakes has been addressed in several cases.
Coastal waters
[edit]-
Map of measured Gulf hypoxia zone, July 25–31, 2021, LUMCON-NOAA
-
Oxygen minimum zones (OMZs) (blue) and areas with coastal hypoxia (red) in the world's ocean[52]
Eutrophication is a common phenomenon in coastal waters, where nitrogenous sources are the main culprit.[21] In coastal waters, nitrogen is commonly the key limiting nutrient of marine waters (unlike the freshwater systems where phosphorus is often the limiting nutrient). Therefore, nitrogen levels are more important than phosphorus levels for understanding and controlling eutrophication problems in salt water.[53] Estuaries, as the interface between freshwater and saltwater, can be both phosphorus and nitrogen limited and commonly exhibit symptoms of eutrophication. Eutrophication in estuaries often results in bottom water hypoxia or anoxia, leading to fish kills and habitat degradation.[54] Upwelling in coastal systems also promotes increased productivity by conveying deep, nutrient-rich waters to the surface, where the nutrients can be assimilated by algae.
Examples of anthropogenic sources of nitrogen-rich pollution to coastal waters include sea cage fish farming and discharges of ammonia from the production of coke from coal.[55] In addition to runoff from land, wastes from fish farming and industrial ammonia discharges, atmospheric fixed nitrogen can be an important nutrient source in the open ocean. This could account for around one third of the ocean's external (non-recycled) nitrogen supply, and up to 3% of the annual new marine biological production.[56]
Coastal waters embrace a wide range of marine habitats from enclosed estuaries to the open waters of the continental shelf. Phytoplankton productivity in coastal waters depends on both nutrient and light supply, with the latter an important limiting factor in waters near to shore where sediment resuspension often limits light penetration.
Nutrients are supplied to coastal waters from land via river and groundwater and also via the atmosphere. There is also an important source from the open ocean, via mixing of relatively nutrient rich deep ocean waters.[57] Nutrient inputs from the ocean are little changed by human activity, although climate change may alter the water flows across the shelf break. By contrast, inputs from land to coastal zones of the nutrients nitrogen and phosphorus have been increased by human activity globally. The extent of increases varies greatly from place to place depending on human activities in the catchments.[58][59] A third key nutrient, dissolved silicon, is derived primarily from sediment weathering to rivers and from offshore and is therefore much less affected by human activity.
Effects of coastal eutrophication
[edit]These increasing nitrogen and phosphorus nutrient inputs exert eutrophication pressures on coastal zones. These pressures vary geographically depending on the catchment activities and associated nutrient load. The geographical setting of the coastal zone is another important factor as it controls dilution of the nutrient load and oxygen exchange with the atmosphere. The effects of these eutrophication pressures can be seen in several different ways:
- There is evidence from satellite monitoring that the amounts of chlorophyll as a measure of overall phytoplankton activity are increasing in many coastal areas worldwide due to increased nutrient inputs.[60]
- The phytoplankton species composition may change due to increased nutrient loadings and changes in the proportions of key nutrients. In particular the increases in nitrogen and phosphorus inputs, along with much smaller changes in silicon inputs, create changes in the ratio of nitrogen and phosphorus to silicon. These changing nutrient ratios drive changes in phytoplankton species composition, particularly disadvantaging silica rich phytoplankton species like diatoms compared to other species.[57] This process leads to the development of nuisance algal blooms in areas such as the North Sea[61] (see also OSPAR Convention) and the Black Sea.[62] In some cases nutrient enrichment can lead to harmful algal blooms (HABs). Such blooms can occur naturally, but there is good evidence that these are increasing as a result of nutrient enrichment, although the causal linkage between nutrient enrichment and HABs is not straightforward.[63]
- Oxygen depletion has existed in some coastal seas such as the Baltic for thousands of years. In such areas the density structure of the water column severely restricts water column mixing and associated oxygenation of deep water. However, increases in the inputs of bacterially degradable organic matter to such isolated deep waters can exacerbate such oxygen depletion in oceans. These areas of lower dissolved oxygen have increased globally in recent decades. They are usually connected with nutrient enrichment and resulting algal blooms.[52] Climate change will generally tend to increase water column stratification and so exacerbate this oxygen depletion problem.[64] An example of such coastal oxygen depletion is in the Gulf of Mexico where an area of seasonal anoxia more than 5000 square miles in area has developed since the 1950s. The increased primary production driving this anoxia is fueled by nutrients supplied by the Mississippi river.[65] A similar process has been documented in the Black Sea.[62]
- Hypolimnetic oxygen depletion can lead to summer "kills". During summer stratification, inputs or organic matter and sedimentation of primary producers can increase rates of respiration in the hypolimnion. If oxygen depletion becomes extreme, aerobic organisms (such as fish) may die, resulting in what is known as a "summer kill".[66]
Extent of the problem
[edit]Surveys showed that 54% of lakes in Asia are eutrophic; in Europe, 53%; in North America, 48%; in South America, 41%; and in Africa, 28%.[67] In South Africa, a study by the CSIR using remote sensing has shown more than 60% of the reservoirs surveyed were eutrophic.[68]
The World Resources Institute has identified 375 hypoxic coastal zones in the world, concentrated in coastal areas in Western Europe, the Eastern and Southern coasts of the US, and East Asia, particularly Japan.[69]
Prevention
[edit]As a society, there are certain steps we can take to ensure the minimization of eutrophication, thereby reducing its harmful effects on humans and other living organisms in order to sustain a healthy norm of living, some of which are as follows:
Minimizing pollution from sewage
[edit]There are multiple different ways to fix cultural eutrophication with raw sewage being a point source of pollution. For example, sewage treatment plants can be upgraded for biological nutrient removal so that they discharge much less nitrogen and phosphorus to the receiving water body. However, even with good secondary treatment, most final effluents from sewage treatment works contain substantial concentrations of nitrogen as nitrate, nitrite or ammonia. Removal of these nutrients is an expensive and often difficult process.
Laws regulating the discharge and treatment of sewage have led to dramatic nutrient reductions to surrounding ecosystems.[70] As a major contributor to the nonpoint source nutrient loading of water bodies is untreated domestic sewage, it is necessary to provide treatment facilities to highly urbanized areas, particularly those in developing countries, in which treatment of domestic waste water is a scarcity. The technology to safely and efficiently reuse wastewater, both from domestic and industrial sources, should be a primary concern for policy regarding eutrophication.
Minimizing nutrient pollution by agriculture
[edit]There are many ways to help fix cultural eutrophication caused by agriculture. Some recommendations issued by the U.S. Department of Agriculture include:[71]
- Nutrient management techniques - Anyone using fertilizers should apply fertilizer in the correct amount, at the right time of year, with the right method and placement. Organically fertilized fields can "significantly reduce harmful nitrate leaching" compared to conventionally fertilized fields.[72] Eutrophication impacts are in some cases higher from organic production than they are from conventional production.[73] In Japan the amount of nitrogen produced by livestock is adequate to serve the fertilizer needs for the agriculture industry.[74]
- Year-round ground cover - a cover crop will prevent periods of bare ground thus eliminating erosion and runoff of nutrients even after the growing season has passed.
- Planting field buffers - Planting trees, shrubs and grasses along the edges of fields can help catch the runoff and absorb some nutrients before the water makes it to a nearby water body.[75] Riparian buffer zones are interfaces between a flowing body of water and land, and have been created near waterways in an attempt to filter pollutants; sediment and nutrients are deposited here instead of in water. Creating buffer zones near farms and roads is another possible way to prevent nutrients from traveling too far.
- Conservation tillage - By reducing frequency and intensity of tilling, the land will enhance the chance of nutrients absorbing into the ground.

Policy
[edit]The United Nations framework for Sustainable Development Goals recognizes the damaging effects of eutrophication for marine environments. It has established a timeline for creating an Index of Coastal Eutrophication and Floating Plastic Debris Density (ICEP) within Sustainable Development Goal 14 (life below water).[76] SDG 14 specifically has a target to: "by 2025, prevent and significantly reduce marine pollution of all kinds, in particular from land-based activities, including marine debris and nutrient pollution".[77]
Policy and regulations are a set of tools to minimize causes of eutrophication.[78] Nonpoint sources of pollution are the primary contributors to eutrophication, and their effects can be minimized through common agricultural practices. Reducing the amount of pollutants that reach a watershed can be achieved through the protection of its forest cover, reducing the amount of erosion leeching into a watershed. Also, through the efficient, controlled use of land using sustainable agricultural practices to minimize land degradation, the amount of soil runoff and nitrogen-based fertilizers reaching a watershed can be reduced.[79] Waste disposal technology constitutes another factor in eutrophication prevention.
Because a body of water can have an effect on a range of people reaching far beyond that of the watershed, cooperation between different organizations is necessary to prevent the intrusion of contaminants that can lead to eutrophication. Agencies ranging from state governments to those of water resource management and non-governmental organizations, going as low as the local population, are responsible for preventing eutrophication of water bodies. In the United States, the most well known inter-state effort to prevent eutrophication is the Chesapeake Bay.[80]
Reversal and remediation
[edit]Reducing nutrient inputs is a crucial precondition for restoration. Still, there are two caveats: Firstly, it can take a long time, mainly because of the storage of nutrients in sediments. Secondly, restoration may need more than a simple reversal of inputs since there are sometimes several stable but very different ecological states.[81] Recovery of eutrophicated lakes is slow, often requiring several decades.[18]
In environmental remediation, nutrient removal technologies include biofiltration, which uses living material to capture and biologically degrade pollutants. Examples include green belts, riparian areas, natural and constructed wetlands, and treatment ponds.
Algae bloom forecasting
[edit]The National Oceanic Atmospheric Admiration in the United States has created a forecasting tool for regions such as the Great Lakes, the Gulf of Maine, and The Gulf of Mexico.[82] Shorter term predictions can help to show the intensity, location, and trajectory of blooms in order to warn more directly affected communities. Longer term tests in specific regions and bodies help to predict larger scale factors like scale of future blooms and factors that could lead to more adverse effects.[83]
Nutrient bioextraction
[edit]Nutrient bioextraction is bioremediation involving cultured plants and animals. Nutrient bioextraction or bioharvesting is the practice of farming and harvesting shellfish and seaweed to remove nitrogen and other nutrients from natural water bodies.[84]
Shellfish in estuaries
[edit]It has been suggested that nitrogen removal by oyster reefs could generate net benefits for sources facing nitrogen emission restrictions, similar to other nutrient trading scenarios. Specifically, if oysters maintain nitrogen levels in estuaries below thresholds, then oysters effectively stave off an enforcement response, and compliance costs parties responsible for nitrogen emission would otherwise incur.[85] Several studies have shown that oysters and mussels can dramatically impact nitrogen levels in estuaries.[86][87][88] Filter feeding activity is considered beneficial to water quality[89] by controlling phytoplankton density and sequestering nutrients, which can be removed from the system through shellfish harvest, buried in the sediments, or lost through denitrification.[90][91] Foundational work toward the idea of improving marine water quality through shellfish cultivation was conducted by Odd Lindahl et al., using mussels in Sweden.[92] In the United States, shellfish restoration projects have been conducted on the East, West and Gulf coasts.[93]
Seaweed farming
[edit]Studies have demonstrated seaweed's potential to improve nitrogen levels.[94][95] Seaweed aquaculture offers an opportunity to mitigate, and adapt to climate change.[96] Seaweed, such as kelp, also absorbs phosphorus and nitrogen[97] and is thus helpful to remove excessive nutrients from polluted parts of the sea.[98] Some cultivated seaweeds have very high productivity and could absorb large quantities of N, P, CO2, producing large amounts of O2 having an excellent effect on decreasing eutrophication.[99] It is believed that seaweed cultivation in large scale should be a good solution to the eutrophication problem in coastal waters.
Geo-engineering
[edit]
Another technique for combatting hypoxia/eutrophication in localized situations is direct injection of compressed air, a technique used in the restoration of the Salford Docks area of the Manchester Ship Canal in England.[100] For smaller-scale waters such as aquaculture ponds, pump aeration is standard.[101]
Chemical removal of phosphorus
[edit]Removing phosphorus can remediate eutrophication.[102][103] Of the several phosphate sorbents, alum (aluminium sulfate) is of practical interest.[104]) Many materials have been investigated.[105][106] The phosphate sorbent is commonly applied in the surface of the water body and it sinks to the bottom of the lake reducing phosphate, such sorbents have been applied worldwide to manage eutrophication and algal bloom (for example under the commercial name Phoslock).[107][108][109][110][111] In a large-scale study, 114 lakes were monitored for the effectiveness of alum at phosphorus reduction. Across all lakes, alum effectively reduced the phosphorus for 11 years. While there was variety in longevity (21 years in deep lakes and 5.7 years in shallow lakes), the results express the effectiveness of alum at controlling phosphorus within lakes.[112] Alum treatment is less effective in deep lakes, as well as lakes with substantial external phosphorus loading.[113]
Finnish phosphorus removal measures started in the mid-1970s and have targeted rivers and lakes polluted by industrial and municipal discharges. These efforts have had a 90% removal efficiency.[114] Still, some targeted point sources did not show a decrease in runoff despite reduction efforts.
See also
[edit]- Biogeochemical cycle – Chemical transfer pathway between Earth's biological and non-biological parts
- Ecological Quality Ratio – Directive
- Effluent – Liquid waste or sewage discharged into a river or the sea
- Nitrogen cycle – Biogeochemical cycle by which nitrogen is converted into various chemical forms
- Trophic state index – Measure of the ability of water to sustain biological productivity
- Upland and lowland (freshwater ecology) – Types of plain
- Water Framework Directive
External links
[edit]
References
[edit]- ^ a b "Nutrients and Eutrophication | U.S. Geological Survey". www.usgs.gov. Retrieved February 9, 2024.
- ^ Aczel MR (2019). "What Is the Nitrogen Cycle and Why Is It Key to Life?". Frontiers for Young Minds. 7. doi:10.3389/frym.2019.00041. hdl:10044/1/71039.
- ^ "Cultural eutrophication | ecology | Britannica". www.britannica.com. Retrieved February 9, 2024.
- ^ Carpenter SR (2008). "Phosphorus control is critical to mitigating eutrophication". Proceedings of the National Academy of Sciences. 105 (32): 11039–11040. Bibcode:2008PNAS..10511039C. doi:10.1073/pnas.0806112105. PMC 2516213. PMID 18685114.
- ^ "Eutrophication and Oyster Aquaculture in the Potomac River Estuary". NCCOS Coastal Science Website. Retrieved February 9, 2024.
- ^ "eutrophia", American Heritage Dictionary of the English Language (Fifth ed.), Houghton Mifflin Harcourt Publishing Company, 2016, archived from the original on March 11, 2018, retrieved March 10, 2018
- ^ Wetzel R (1975). Limnology. Philadelphia-London-Toronto: W.B. Saunders. p. 743. ISBN 0-7216-9240-0.
- ^ Smil V. "Nitrogen Cycle and World Food Production" (PDF).
- ^ Rodhe, W. (1969) "Crystallization of Eutrophication Concepts in North Europe". In: Eutrophication, Causes, Consequences, Correctives. National Academy of Sciences, Washington D.C., ISBN 9780309017008 , pp. 50–64.
- ^ Schindler D (1974). "Eutrophication and Recovery in Experimental Lakes: Implications for Lake Management". Science. 184 (4139) (4139): 897–899. Bibcode:1974Sci...184..897S. doi:10.1126/science.184.4139.897. PMID 17782381. S2CID 25620329.
- ^ Schindler, David and Vallentyne, John R. (2004) Over fertilization of the World's Freshwaters and Estuaries, University of Alberta Press, p. 1, ISBN 0-88864-484-1
- ^ Werner, Wilfried (2002) "Fertilizers, 6. Environmental Aspects". Ullmann's Encyclopedia of Industrial Biology, Wiley-VCH, Weinheim. doi:10.1002/14356007.n10_n05
- ^ Fowler D, Coyle M, Skiba U, Sutton MA, Cape JN, Reis S, Sheppard LJ, Jenkins A, Grizzetti B, Galloway JN, Vitousek P (2013). "The global nitrogen cycle in the twenty-first century". Philosophical Transactions of the Royal Society B: Biological Sciences. 368 (1621): 20130164. doi:10.1098/rstb.2013.0164. PMC 3682748. PMID 23713126.
- ^ Moore CM, Mills MM, Arrigo KR, Berman-Frank I, Bopp L, Boyd PW, Galbraith ED, Geider RJ, Guieu C, Jaccard SL, Jickells TD, La Roche J, Lenton TM, Mahowald NM, Marañón E (September 2013). "Processes and patterns of oceanic nutrient limitation". Nature Geoscience. 6 (9): 701–710. Bibcode:2013NatGe...6..701M. doi:10.1038/ngeo1765. ISSN 1752-0908. S2CID 249514.
- ^ Elser JJ, Bracken ME, Cleland EE, Gruner DS, Harpole WS, Hillebrand H, Ngai JT, Seabloom EW, Shurin JB, Smith JE (July 2007). "Global analysis of nitrogen and phosphorus limitation of primary producers in freshwater, marine and terrestrial ecosystems". Ecology Letters. 10 (12): 1135–1142. Bibcode:2007EcolL..10.1135E. doi:10.1111/j.1461-0248.2007.01113.x. hdl:1903/7447. ISSN 1461-023X. PMID 17922835. S2CID 12083235.
- ^ "Phosphorus Basics: Understanding Phosphorus Forms and Their Cycling in the Soil". Alabama Cooperative Extension System. Retrieved February 10, 2024.
- ^ US EPA OW (November 27, 2013). "Indicators: Phosphorus". www.epa.gov. Retrieved February 10, 2024.
- ^ a b Schindler DW (2012). "The dilemma of controlling cultural eutrophication of lakes". Proceedings of the Royal Society B: Biological Sciences. 279 (1746): 4322–4333. doi:10.1098/rspb.2012.1032. PMC 3479793. PMID 22915669.
- ^ Reay D (November 9, 2002). "Nitrous oxide Sources - Oceans". ghgonline. Archived from the original on December 7, 2023. Retrieved February 11, 2024.
- ^ Bristow L, Mohr W (2017). "Nutrients that limit growth in the ocean". Current Biology. 27 (11): R431–R510. Bibcode:2017CBio...27.R474B. doi:10.1016/j.cub.2017.03.030. hdl:21.11116/0000-0001-C1AA-5. PMID 28586682. S2CID 21052483. Archived from the original on September 28, 2022. Retrieved June 17, 2021.
- ^ a b c Smith VH, Schindler DW (2009). "Eutrophication science: Where do we go from here?". Trends in Ecology & Evolution. 24 (4): 201–207. doi:10.1016/j.tree.2008.11.009. PMID 19246117.
- ^ Cultural eutrophication Archived May 4, 2015, at the Wayback Machine (2010) Encyclopedia Britannica. Retrieved April 26, 2010, from Encyclopedia Britannica Online:
- ^ Smil V (November 2000). "Phosphorus in the Environment: Natural Flows and Human Interferences". Annual Review of Energy and the Environment. 25 (1): 53–88. doi:10.1146/annurev.energy.25.1.53. ISSN 1056-3466.
- ^ a b Moss B (1983). "The Norfolk Broadland: Experiments in the Restoration of a Complex Wetland". Biological Reviews. 58 (4): 521–561. doi:10.1111/j.1469-185X.1983.tb00399.x. ISSN 1469-185X. S2CID 83803387. Archived from the original on February 8, 2022. Retrieved February 8, 2022.
- ^ a b Rabalais NN (March 2002). "Nitrogen in aquatic ecosystems". Ambio: A Journal of the Human Environment. 31 (2): 102–112. Bibcode:2002Ambio..31..102R. doi:10.1579/0044-7447-31.2.102. PMID 12077998. S2CID 19172194.
- ^ Schindler, David W., Vallentyne, John R. (2008). The Algal Bowl: Overfertilization of the World's Freshwaters and Estuaries, University of Alberta Press, ISBN 0-88864-484-1.
- ^ Qin B, Yang L, Chen F, Zhu G, Zhang L, Chen Y (October 1, 2006). "Mechanism and control of lake eutrophication". Chinese Science Bulletin. 51 (19): 2401–2412. Bibcode:2006ChSBu..51.2401Q. doi:10.1007/s11434-006-2096-y. ISSN 1861-9541. S2CID 198137333.
- ^ Khan MN, Mohammad F (2014), Ansari AA, Gill SS (eds.), "Eutrophication: Challenges and Solutions", Eutrophication: Causes, Consequences and Control: Volume 2, Springer Netherlands, pp. 1–15, doi:10.1007/978-94-007-7814-6_1, ISBN 978-94-007-7814-6
- ^ Clair N. Sawyer (May 1966). "Basic Concepts of Eutrophication". Journal (Water Pollution Control Federation). 38 (5). Wiley: 737–744. JSTOR 25035549. Archived from the original on June 3, 2021. Retrieved February 12, 2021.
- ^ Addy K (1996). "Phosphorus and Lake Aging" (PDF). Natural Resources Facts - University of Rhode Island. Archived (PDF) from the original on July 28, 2021. Retrieved June 16, 2021.
- ^ Wetzel RG (2001). Limnology: lake and river ecosystems (3rd ed.). San Diego: Academic Press. ISBN 0-12-744760-1. OCLC 46393244. Archived from the original on November 2, 2020. Retrieved February 8, 2022.
- ^ Walker, I. R. (2006) "Chironomid overview", pp. 360–366 in S.A. EIias (ed.) Encyclopedia of Quaternary Science, Vol. 1, Elsevier,
- ^ Whiteside MC (1983). "The mythical concept of eutrophication". Hydrobiologia. 103: 107–150. doi:10.1007/BF00028437. S2CID 19039247.
- ^ Callisto, Marcos; Molozzi, Joseline and Barbosa, José Lucena Etham (2014) "Eutrophication of Lakes" in A. A. Ansari, S. S. Gill (eds.), Eutrophication: Causes, Consequences and Control, Springer Science+Business Media Dordrecht. doi:10.1007/978-94-007-7814-6_5. ISBN 978-94-007-7814-6.
- ^ "Nutrients and Eutrophication | U.S. Geological Survey". www.usgs.gov. Retrieved September 29, 2024.
- ^ Horrigan L, Lawrence RS, Walker P (2002). "How sustainable agriculture can address the environmental and human health harms of industrial agriculture". Environmental Health Perspectives. 110 (5): 445–456. doi:10.1289/ehp.02110445. PMC 1240832. PMID 12003747.
- ^ Bertness MD, Ewanchuk PJ, Silliman BR (2002). "Anthropogenic modification of New England salt marsh landscapes". Proceedings of the National Academy of Sciences of the United States of America. 99 (3): 1395–1398. Bibcode:2002PNAS...99.1395B. doi:10.1073/pnas.022447299. JSTOR 3057772. PMC 122201. PMID 11818525.
- ^ Anderson D. M. (1994). "Red tides" (PDF). Scientific American. 271 (2): 62–68. Bibcode:1994SciAm.271b..62A. doi:10.1038/scientificamerican0894-62. PMID 8066432. Archived (PDF) from the original on May 11, 2013. Retrieved March 31, 2013.
- ^ Lawton L, G.A. Codd (1991). "Cyanobacterial (blue-green algae) toxins and their significance in UK and European waters". Journal of Soil and Water Conservation. 40 (4): 87–97. doi:10.1111/j.1747-6593.1991.tb00643.x.
- ^ Martin A, G.D. Cooke (1994). "Health risks in eutrophic water supplies". Lake Line. 14: 24–26.
- ^ Shumway SE (1990). "A Review of the Effects of Algal Blooms on Shellfish and Aquaculture". Journal of the World Aquaculture Society. 21 (2): 65–104. Bibcode:1990JWAS...21...65S. doi:10.1111/j.1749-7345.1990.tb00529.x.
- ^ US EPA OW (2013). "The Effects: Economy". www.epa.gov. Archived from the original on September 28, 2022. Retrieved February 15, 2022.
- ^ Xiao (June 2, 2017). "Water Eutrophication and its Effect • EnvGuide". EnvGuide. Retrieved October 28, 2024.
- ^ "The Effects: Human Health". Nutrient Pollution. EPA. March 1, 2021. Archived from the original on February 19, 2020. Retrieved February 21, 2022.
- ^ US EPA OW (2013). "The Effects: Human Health". www.epa.gov. Archived from the original on February 19, 2020. Retrieved February 15, 2022.
- ^ Schindler DW, Hecky R, Findlay D, Stainton M, Parker B, Paterson M, Beaty K, Lyng M, Kasian SE (August 2008). "Eutrophication of lakes cannot be controlled by reducing nitrogen input: Results of a 37-year whole-ecosystem experiment". Proceedings of the National Academy of Sciences of the United States of America. 105 (32): 11254–11258. doi:10.1073/pnas.0805108105. PMC 2491484. PMID 18667696.
- ^ "Climate gases from water bodies". Archived from the original on February 2, 2019. Retrieved September 22, 2018.
- ^ "Nature's Value Chain..." (PDF). Archived from the original (PDF) on December 21, 2016. Retrieved September 22, 2018.
- ^ Jeppesen E, Søndergaard M, Jensen JP, Havens KE, Anneville O, Carvalho L, Coveney MF, Deneke R, Dokulil MT, Foy B, Gerdeaux D (2005). "Lake responses to reduced nutrient loading – an analysis of contemporary long-term data from 35 case studies". Freshwater Biology. 50 (10): 1747–1771. Bibcode:2005FrBio..50.1747J. doi:10.1111/j.1365-2427.2005.01415.x. ISSN 1365-2427.
- ^ Bartram, J., Wayne W. Carmichael, Ingrid Chorus, Gary Jones, and Olav M. Skulberg (1999). "Chapter 1. Introduction", in: Toxic Cyanobacteria in Water: A guide to their public health consequences, monitoring and management. World Health Organization. URL: WHO document Archived January 24, 2007, at the Wayback Machine
- ^ Higgins SN, Paterson MJ, Hecky RE, Schindler DW, Venkiteswaran JJ, Findlay DL (November 27, 2017). "Biological Nitrogen Fixation Prevents the Response of a Eutrophic Lake to Reduced Loading of Nitrogen: Evidence from a 46-Year Whole-Lake Experiment". Ecosystems. 21 (6): 1088–1100. doi:10.1007/s10021-017-0204-2. S2CID 26030685.
- ^ a b Breitburg D, Levin LA, Oschlies A, Grégoire M, Chavez FP, Conley DJ, Garçon V, Gilbert D, Gutiérrez D, Isensee K, Jacinto GS (2018). "Declining oxygen in the global ocean and coastal waters". Science. 359 (6371). Bibcode:2018Sci...359M7240B. doi:10.1126/science.aam7240. PMID 29301986. S2CID 206657115.
- ^ Paerl HW, Valdes LM, Joyner AR, Piehler MF, Lebo ME (2004). "Solving problems resulting from solutions: Evolution of a dual nutrient management strategy for the eutrophying Neuse River Estuary, North Carolina". Environmental Science and Technology. 38 (11): 3068–3073. Bibcode:2004EnST...38.3068P. doi:10.1021/es0352350. PMID 15224737.
- ^ Huang J, Xu Cc, Ridoutt B, Wang Xc, Ren Pa (August 2017). "Nitrogen and phosphorus losses and eutrophication potential associated with fertilizer application to cropland in China". Journal of Cleaner Production. 159: 171–179. doi:10.1016/j.jclepro.2017.05.008.
- ^ "Recovery of Ammonia during Production of Coke from Coking Coal". Ispat Guru. 2019. Archived from the original on June 24, 2021. Retrieved June 17, 2021.
- ^ Duce RA, et al. (2008). "Impacts of Atmospheric Anthropogenic Nitrogen on the Open Ocean". Science. 320 (5878): 893–89. Bibcode:2008Sci...320..893D. doi:10.1126/science.1150369. hdl:21.11116/0000-0001-CD7A-0. PMID 18487184. S2CID 11204131.
- ^ a b Jickells TD (1998). "Nutrient Biogeochemistry of the Coastal Zone". Science. 281 (5374): 217–222. doi:10.1126/science.281.5374.217. ISSN 0036-8075. PMID 9660744.
- ^ Seitzinger SP, Mayorga E, Bouwman AF, Kroeze C, Beusen AH, Billen G, Van Drecht G, Dumont E, Fekete BM, Garnier J, Harrison JA (2010). "Global river nutrient export: A scenario analysis of past and future trends: GLOBAL RIVER EXPORT SCENARIOS". Global Biogeochemical Cycles. 24 (4): n/a. doi:10.1029/2009GB003587. S2CID 55095122.
- ^ Jickells TD, Buitenhuis E, Altieri K, Baker AR, Capone D, Duce RA, Dentener F, Fennel K, Kanakidou M, LaRoche J, Lee K (2017). "A reevaluation of the magnitude and impacts of anthropogenic atmospheric nitrogen inputs on the ocean: Atmospheric nitrogen inputs". Global Biogeochemical Cycles. 31 (2): 289–305. doi:10.1002/2016GB005586. hdl:1874/348077. S2CID 5158406.
- ^ Maúre Ed, Terauchi G, Ishizaka J, Clinton N, DeWitt M (2021). "Globally consistent assessment of coastal eutrophication". Nature Communications. 12 (1): 6142. doi:10.1038/s41467-021-26391-9. ISSN 2041-1723. PMC 8536747. PMID 34686688.
- ^ Ltd MC. "Intermediate Assessment 2017". oap.ospar.org. Archived from the original on February 9, 2022. Retrieved February 9, 2022.
- ^ a b Mee L, Friedrich J, Gomoiu M (2005). "Restoring the Black Sea in Times of Uncertainty". Oceanography. 18 (2): 100–111. doi:10.5670/oceanog.2005.45. ISSN 1042-8275.
- ^ Glibert P, Burford M (2017). "Globally Changing Nutrient Loads and Harmful Algal Blooms: Recent Advances, New Paradigms, and Continuing Challenges". Oceanography. 30 (1): 58–69. doi:10.5670/oceanog.2017.110. hdl:10072/377577. Archived from the original on January 21, 2022. Retrieved February 9, 2022.
- ^ Li G, Cheng L, Zhu J, Trenberth KE, Mann ME, Abraham JP (2020). "Increasing ocean stratification over the past half-century". Nature Climate Change. 10 (12): 1116–1123. Bibcode:2020NatCC..10.1116L. doi:10.1038/s41558-020-00918-2. ISSN 1758-678X. S2CID 221985871. Archived from the original on February 18, 2022. Retrieved February 18, 2022.
- ^ Rabalais NN, Turner RE (2019). "Gulf of Mexico Hypoxia: Past, Present, and Future". Limnology and Oceanography Bulletin. 28 (4): 117–124. Bibcode:2019LimOB..28..117R. doi:10.1002/lob.10351. ISSN 1539-6088. S2CID 209578424.
- ^ Wetzel, R. G. (2001). Limnology: Lake and river ecosystems. San Diego: Academic Press.
- ^ ILEC/Lake Biwa Research Institute [Eds]. 1988–1993 Survey of the State of the World's Lakes. Volumes I-IV. International Lake Environment Committee, Otsu and United Nations Environment Programme, Nairobi.
- ^ Matthews M, Bernard S (2015). "Eutrophication and cyanobacteria in South Africa's standing water bodies: A view from space". South African Journal of Science. 111 (5/6): 1–8. doi:10.17159/sajs.2015/20140193.
- ^ Selman, Mindy (2007) Eutrophication: An Overview of Status, Trends, Policies, and Strategies. World Resources Institute.
- ^ Smith VH, Tilman GD, Nekola JC (1999). "Eutrophication: Impacts of excess nutrient inputs on freshwater, marine, and terrestrial ecosystems". Environmental Pollution. 100 (1–3): 179–196. doi:10.1016/S0269-7491(99)00091-3. PMID 15093117. S2CID 969039.
- ^ "The Sources and Solutions: Agriculture". United States EPA. March 12, 2013. Archived from the original on June 22, 2021.
- ^ Kramer SB (2006). "Reduced nitrate leaching and enhanced denitrifier activity and efficiency in organically fertilized soils". Proceedings of the National Academy of Sciences. 103 (12): 4522–4527. Bibcode:2006PNAS..103.4522K. doi:10.1073/pnas.0600359103. PMC 1450204. PMID 16537377.
- ^ Williams, A.G., Audsley, E. and Sandars, D.L. (2006) Determining the environmental burdens and resource use in the production of agricultural and horticultural commodities Archived September 25, 2018, at the Wayback Machine. Main Report. Defra Research Project IS0205. Bedford: Cranfield University and Defra.
- ^ Kumazawa K (2002). "Nitrogen fertilization and nitrate pollution in groundwater in Japan: Present status and measures for sustainable agriculture". Nutrient Cycling in Agroecosystems. 63 (2/3): 129–137. doi:10.1023/A:1021198721003. S2CID 22847510.
- ^ Carpenter SR, Caraco NF, Correll DL, Howarth RW, Sharpley AN, Smith VH (August 1998). "Nonpoint Pollution of Surface Waters with Phosphorus and Nitrogen". Ecological Applications. 8 (3): 559. doi:10.2307/2641247. hdl:1813/60811. JSTOR 2641247.
- ^ "14.1.1 Index of Coastal Eutrophication (ICEP) and Floating Plastic debris Density". UN Environment. Archived from the original on August 13, 2020. Retrieved October 14, 2020.
- ^ "Goal 14 targets". UNDP. Archived from the original on September 30, 2020. Retrieved September 24, 2020.
- ^ "Planning and Management of Lakes and Reservoirs: An Integrated Approach to Eutrophication." Archived November 11, 2012, at the Wayback Machine United Nations Environment Programme, Newsletter and Technical Publications. International Environmental Technology Centre. Ch.3.4 (2000).
- ^ Oglesby, R. T., Edmondson, W. T. (1966). "Control of Eutrophication". Journal (Water Pollution Control Federation). 38 (9): 1452–1460. JSTOR 25035632.
- ^ Nutrient Limitation. Department of Natural Resources, Maryland, U.S.
- ^ May L, Olszewska J, Gunn ID, Meis S, Spears BM (2020). "Eutrophication and restoration in temperate lakes". IOP Conference Series: Earth and Environmental Science. 535 (1): 012001. Bibcode:2020E&ES..535a2001M. doi:10.1088/1755-1315/535/1/012001. ISSN 1755-1307. S2CID 225481650.
- ^ "Lake Erie Harmful Algal Bloom Forecast". NCCOS. NOAA. Retrieved February 12, 2024.
- ^ "HAB Forecasts". NCCOS Coastal Science Website. Retrieved November 4, 2024.
- ^ "Nutrient Bioextraction Overview". Stamford, CT: Long Island Sound Study partnership. Archived from the original on October 6, 2017. Retrieved March 22, 2018.
- ^ Kroeger T (2012). "Dollars and Sense: Economic Benefits and Impacts from two Oyster Reef Restoration Projects in the Northern Gulf of Mexico" (PDF). The Nature Conservancy. Archived from the original (PDF) on March 4, 2016. Retrieved May 29, 2013.
- ^ Newell RI, Fisher TR, Holyoke RR, Cornwell JC (2005). "Influence of eastern oysters on nitrogen and phosphorus regeneration in Chesapeake Bay, USA". In Dame R, Olenin S (eds.). The Comparative Roles of Suspension Feeders in Ecosystems. Vol. 47 (NATO Science Series IV: Earth and Environmental Sciences ed.). Netherlands: Springer. pp. 93–120.
- ^ Grabowski JH, Petersen CM (2007). Cuddington K, Byers JE, Wilson WG, Hastings A (eds.). Restoring oyster reefs to recover ecosystem services (Ecosystem Engineers: Concepts, Theory and Applications ed.). Amsterdam: Elsevier-Academic Press. pp. 281–298.
- ^ Rose JM, Tedesco M, Wikfors GH, Yarish C (2010). "International Workshop on Bioextractive Technologies for Nutrient Remediation Summary Report". US Dept Commerce, Northeast Fish Sci Cent Ref Doc. 10-19; 12 p. Available from: National Marine Fisheries Service, 166 Water Street, Woods Hole, MA 02543-1026. Archived from the original on October 29, 2019. Retrieved February 15, 2022.
- ^ Burkholder, JoAnn M. and Sandra E. Shumway. (2011) "Bivalve shellfish aquaculture and eutrophication", in Shellfish Aquaculture and the Environment. Ed. Sandra E. Shumway. John Wiley & Sons, ISBN 0-8138-1413-8.
- ^ Kaspar HF, Gillespie PA, Boyer IC, MacKenzie AL (1985). "Effects of mussel aquaculture on the nitrogen cycle and benthic communities in Kenepuru Sound, Marlborough Sounds, New Zealand". Marine Biology. 85 (2): 127–136. Bibcode:1985MarBi..85..127K. doi:10.1007/BF00397431. S2CID 83551118.
- ^ Newell RI, Cornwell JC, Owens MS (2002). "Influence of simulated bivalve biodeposition and microphytobenthos on sediment nitrogen dynamics: A laboratory study". Limnology and Oceanography. 47 (5): 1367–1379. Bibcode:2002LimOc..47.1367N. doi:10.4319/lo.2002.47.5.1367.
- ^ Lindahl O, Hart R, Hernroth B, Kollberg S, Loo LO, Olrog L, Rehnstam-Holm AS, Svensson J, Svensson S, Syversen U (2005). "Improving marine water quality by mussel farming: A profitable solution for Swedish society" (PDF). Ambio. 34 (2): 131–138. Bibcode:2005Ambio..34..131L. CiteSeerX 10.1.1.589.3995. doi:10.1579/0044-7447-34.2.131. PMID 15865310. S2CID 25371433. Archived (PDF) from the original on September 22, 2017. Retrieved November 1, 2017.
- ^ Brumbaugh, R.D. et al. (2006). A Practitioners Guide to the Design and Monitoring of Shellfish Restoration Projects: An Ecosystem Services Approach Archived July 1, 2013, at the Wayback Machine. The Nature Conservancy, Arlington, VA.
- ^ Kim JK, Kraemer GP, Yarish C (2014). "Field scale evaluation of seaweed aquaculture as a nutrient bioextraction strategy in Long Island Sound and the Bronx River Estuary". Aquaculture. 433: 148–156. Bibcode:2014Aquac.433..148K. doi:10.1016/j.aquaculture.2014.05.034.
- ^ Kroeger T (May 2012). "Dollars and Sense: Economic Benefits and Impacts from two Oyster Reef Restoration Projects in the Northern Gulf of Mexico". The Nature Conservancy. Archived from the original on August 3, 2020. Retrieved July 29, 2020.
- ^ Duarte CM, Wu J, Xiao X, Bruhn A, Krause-Jensen D (April 12, 2017). "Can Seaweed Farming Play a Role in Climate Change Mitigation and Adaptation?". Frontiers in Marine Science. 4. doi:10.3389/fmars.2017.00100. hdl:10754/623247.
- ^ "Can We Save the Oceans By Farming Them?". Yale E360. Archived from the original on October 19, 2019. Retrieved March 8, 2019.
- ^ Xiao X, Agusti S, Lin F, Li K, Pan Y, Yu Y, Zheng Y, Wu J, Duarte CM (2017). "Nutrient removal from Chinese coastal waters by large-scale seaweed aquaculture". Scientific Reports. 7: 46613. Bibcode:2017NatSR...746613X. doi:10.1038/srep46613. PMC 5399451. PMID 28429792.
- ^ Duarte CM (2009), "Coastal eutrophication research: A new awareness", Eutrophication in Coastal Ecosystems, Springer Netherlands, pp. 263–269, doi:10.1007/978-90-481-3385-7_22, ISBN 978-90-481-3384-0
- ^ Hindle, P. (August 21, 2003). "Exploring Greater Manchester – a fieldwork guide: The fluvioglacial gravel ridges of Salford and flooding on the River Irwell" (PDF). Manchester Geographical Society. Retrieved December 11, 2007. p. 13
- ^ "Pond Aeration". April 10, 2006.
- ^ Spears BM, Maberly SC, Pan G, MacKay E, Bruere A, Corker N, Douglas G, Egemose S, Hamilton D, Hatton-Ellis T, Huser B, Li W, Meis S, Moss B, Lürling M, Phillips G, Yasseri S, Reitzel K (2014). "Geo-Engineering in Lakes: A Crisis of Confidence?". Environmental Science & Technology. 48 (17): 9977–9979. Bibcode:2014EnST...48.9977S. doi:10.1021/es5036267. PMID 25137490. Archived from the original on October 21, 2021. Retrieved September 8, 2020.
- ^ MacKay E, Maberly S, Pan G, Reitzel K, Bruere A, Corker N, Douglas G, Egemose S, Hamilton D, Hatton-Ellis T, Huser B, Li W, Meis S, Moss B, Lürling M, Phillips G, Yasseri S, Spears B (2014). "Geoengineering in lakes: Welcome attraction or fatal distraction?". Inland Waters. 4 (4): 349–356. Bibcode:2014InWat...4..349M. doi:10.5268/IW-4.4.769. hdl:10072/337267. S2CID 55610343.
- ^ "Wisconsin Department of Natural Resources" (PDF). Archived from the original (PDF) on November 28, 2009. Retrieved August 3, 2010.
- ^ Douglas GB, Hamilton DP, Robb MS, Pan G, Spears BM, Lurling M (2016). "Guiding principles for the development and application of solid-phase phosphorus adsorbents for freshwater ecosystems" (PDF). Aquatic Ecology. 50 (3): 385–405. Bibcode:2016AqEco..50..385D. doi:10.1007/s10452-016-9575-2. hdl:10072/406333. S2CID 18154662. Archived (PDF) from the original on September 19, 2020. Retrieved December 15, 2019.
- ^ Lürling M, MacKay E, Reitzel K, Spears BM (2016). "Editorial – A critical perspective on geo-engineering for eutrophication management in lakes" (PDF). Water Research. 97: 1–10. Bibcode:2016WatRe..97....1L. doi:10.1016/J.WATRES.2016.03.035. PMID 27039034. Archived (PDF) from the original on July 31, 2020. Retrieved December 15, 2019.
- ^ Huser BJ, Egemose S, Harper H, Hupfer M, Jensen H, Pilgrim KM, Reitzel K, Rydin E, Futter M (2016). "Longevity and effectiveness of aluminum addition to reduce sediment phosphorus release and restore lake water quality". Water Research. 97: 122–132. Bibcode:2016WatRe..97..122H. doi:10.1016/j.watres.2015.06.051. PMID 26250754.
- ^ Lürling M, Oosterhout Fv (2013). "Controlling eutrophication by combined bloom precipitation and sediment phosphorus inactivation". Water Research. 47 (17): 6527–6537. Bibcode:2013WatRe..47.6527L. doi:10.1016/j.watres.2013.08.019. PMID 24041525.
- ^ Nürnberg GK (2017). "Attempted management of cyanobacteria by Phoslock (Lanthanum-modified clay) in Canadian lakes: Water quality results and predictions". Lake and Reservoir Management. 33 (2): 163–170. Bibcode:2017LRMan..33..163N. doi:10.1080/10402381.2016.1265618. S2CID 89762486.
- ^ Epe TS, Finsterle K, Yasseri S (2017). "Nine years of phosphorus management with lanthanum modified bentonite (Phoslock) in a eutrophic, shallow swimming lake in Germany". Lake and Reservoir Management. 33 (2): 119–129. Bibcode:2017LRMan..33..119E. doi:10.1080/10402381.2016.1263693. S2CID 90314146.
- ^ Kennedy RH, Cook GD (June 1982). "Control of Lake Phosphorus with Aluminum Sulfate: Dose Determination and Application Techniques". Journal of the American Water Resources Association. 18 (3): 389–395. Bibcode:1982JAWRA..18..389K. doi:10.1111/j.1752-1688.1982.tb00005.x. ISSN 1093-474X.
- ^ Huser BJ, Egemose S, Harper H, Hupfer M, Jensen H, Pilgrim KM, Reitzel K, Rydin E, Futter M (2016). Longevity and effectiveness of aluminum addition to reduce sediment phosphorus release and restore lake water quality. Fjärdingen: Uppsala universitet, Limnologi Uppsala universitet. OCLC 1233676585.
- ^ Cooke, G. D., Welch, E. B., Martin, A. B., Fulmer, D. G., Hyde, J. B., & Schrieve, G. D. (1993). Effectiveness of Al, Ca, and Fe salts for control of internal phosphorus loading in shallow and deep lakes. Hydrobiologia, 253(1), 323-335.
- ^ Räike A, Pietiläinen OP, Rekolainen S, Kauppila P, Pitkänen H, Niemi J, Raateland A, Vuorenmaa J (2003). "Trends of phosphorus, nitrogen and chlorophyll a concentrations in Finnish rivers and lakes in 1975–2000". Science of the Total Environment. 310 (1–3): 47–59. Bibcode:2003ScTEn.310...47R. doi:10.1016/S0048-9697(02)00622-8. PMID 12812730.